The Impact of Fading on Wireless Communication Systems: A Theoretical Discussion
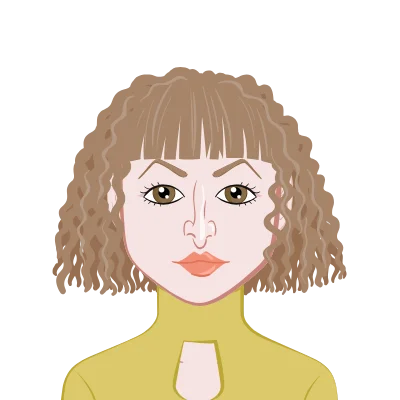
Wireless communication systems have become an integral part of our daily lives, connecting people and devices across the globe. In order to design efficient and robust wireless systems, it is crucial to understand the effects of fading, which can significantly impact the quality and reliability of communication. In this theoretical discussion, we will explore the concept of fading in wireless communication systems, considering different fade models such as Rayleigh, Rician, and Nakagami. If you need assistance with your Wireless communication systems assignment related to fading models or any other aspect of wireless communication, we are here to help. While we won't delve into specific mathematical expressions and code implementations in this discussion, we will provide a comprehensive understanding of the theoretical foundations of fading in wireless communication.
What is Fading in Wireless Communication?
Fading is a phenomenon that occurs in wireless communication when the transmitted signal experiences variations in amplitude, phase, or both, as it propagates through the wireless channel. These variations are primarily caused by multipath propagation, where signals take multiple paths to reach the receiver due to reflections, diffractions, and scattering from objects in the environment. The variations in signal strength and phase introduce challenges in maintaining reliable communication links.
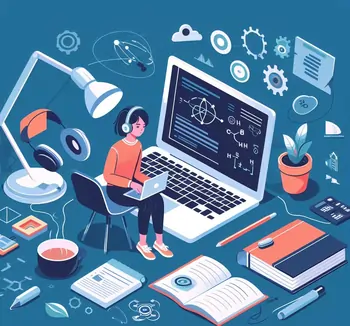
Types of Fading Models
In wireless communication systems, understanding the characteristics of the propagation channel is crucial for designing reliable and efficient communication links. Fading is one of the key phenomena that affect wireless channels, and it can be modeled using various fading models. Among these models, three common ones are Rayleigh fading, Rician fading, and Nakagami fading. In this section, we will delve deeper into each of these fading models to gain a better understanding of their characteristics and applications.
- Rayleigh Fading:
- Magnitude Distribution: In Rayleigh fading, the magnitude of the received signal follows a Rayleigh distribution. This distribution is a special case of the Weibull distribution, which is characterized by its probability density function (PDF) with a single parameter.
- Phase Distribution: The phase of the received signal in Rayleigh fading is uniformly distributed. This means that the phase of the signal is equally likely to take any value between 0 and 2π radians.
- Rician Fading:
- Magnitude Distribution: In Rician fading, the magnitude of the received signal follows a Rician distribution, which is characterized by two parameters: the Rician factor (K) and the LOS amplitude (A). The Rician factor represents the power of the LOS component relative to the multipath components.
- Phase Distribution: Similar to Rayleigh fading, the phase of the received signal in Rician fading is uniformly distributed.
- Nakagami Fading:
- Magnitude Distribution: The magnitude of the received signal in Nakagami fading follows a Nakagami distribution. The Nakagami distribution is a generalization of the Rayleigh distribution, with the Nakagami parameter m playing a crucial role. When m is high, the fading approaches the characteristics of Rayleigh fading, while for m equal to 1, it represents a pure Rayleigh fading channel.
- Versatility: Nakagami fading is versatile because it can describe channel conditions ranging from severe multipath fading (low m values) to nearly non-fading conditions (high m values). This flexibility makes it suitable for modeling a wide variety of wireless environments.
Rayleigh fading is a widely used model to simulate wireless communication channels, particularly in urban and suburban environments. It is characterized by two primary features:
Rayleigh fading typically occurs in scenarios where there is a significant presence of multipath propagation, such as signals bouncing off buildings and other obstacles in urban environments. In this model, there is no dominant line-of-sight (LOS) component, making it suitable for situations where LOS signals are relatively weak.
Rician fading, unlike Rayleigh fading, is used to model communication channels that exhibit both multipath components and a strong LOS component. The key features of Rician fading are:
Rician fading is commonly encountered in scenarios where there is a clear LOS path between the transmitter and receiver, in addition to multipath reflections. Examples of such scenarios include wireless communication links between a mobile device and a base station in an urban area. The Rician factor K quantifies the strength of the LOS component, with higher values indicating a stronger LOS component.
The Nakagami fading model is a versatile fading model that can represent a broad range of channel conditions. It is characterized by the Nakagami parameter (m), which determines the severity of fading. Key characteristics of Nakagami fading include:
Nakagami fading is particularly useful when the channel conditions are not well defined or when a more generalized model is required. The choice of the Nakagami parameter allows engineers to tailor the model to match the specific fading characteristics of a given wireless channel.
Impact of Fading on Communication Systems
Understanding the impact of fading on wireless communication systems is paramount for designing and maintaining robust and reliable communication links. Fading introduces various challenges and considerations that directly affect the performance of these systems. Here, we explore the key aspects of fading's impact on communication systems and the strategies employed to mitigate its effects.
- Signal Degradation:
- Bit Error Rate (BER):
- Diversity Techniques:
- Antenna Diversity: Antenna diversity involves using multiple antennas at the transmitter and/or receiver. By receiving signals through different paths and combining them, antenna diversity can improve signal quality and reduce the impact of fading. Techniques like Maximal Ratio Combining (MRC) and Selection Combining (SC) are commonly used in antenna diversity systems.
- Time Diversity: Time diversity, also known as time-domain diversity, involves transmitting the same information over multiple time instances or symbol periods. By retransmitting data, systems can take advantage of variations in the fading channel over time, reducing the probability of errors and increasing reliability.
- Adaptive Modulation and Coding:
One of the most noticeable effects of fading on communication systems is signal degradation. Fading leads to fluctuations in the received signal's power, causing rapid variations in signal strength. These fluctuations can be so severe that they result in signal loss, dropped calls, and reduced data throughput. Users in a fading-prone environment may experience interruptions in voice calls, slow data transfer rates, or even complete loss of connectivity.
Signal degradation due to fading is especially pronounced in situations where multipath propagation is common, such as urban environments. Tall buildings, vehicles, and other objects in the environment can create multiple paths for the signal to reach the receiver. As a result, signals arriving via different paths may interfere constructively or destructively, leading to fading.
Fading introduces amplitude and phase variations in the received signal, which directly impact the accuracy of data transmission. The Bit Error Rate (BER) is a crucial metric for assessing the performance of communication systems in fading channels. A higher BER indicates a higher likelihood of errors in the received data.
In fading-prone environments, the BER can be significantly elevated, requiring more robust error correction techniques and signal processing to recover the transmitted information accurately. Achieving a low BER in fading channels is challenging but essential for maintaining data integrity and communication quality.
To mitigate the adverse effects of fading, communication systems employ diversity techniques. These techniques are designed to improve signal reliability by leveraging multiple copies of the transmitted signal. Two common diversity techniques are:
In fading channels, it is crucial to adapt the modulation and coding schemes dynamically based on the channel conditions. Different modulation schemes (e.g., QPSK, 16-QAM, 64-QAM) and coding rates (e.g., 1/2, 3/4) provide varying levels of robustness against fading-induced errors.
Adaptive Modulation and Coding (AMC) systems continuously monitor the received signal's quality and adjust the modulation and coding schemes accordingly. When the channel conditions are favorable, higher-order modulation and lower redundancy coding may be used to maximize data throughput. Conversely, in challenging fading conditions, lower-order modulation and higher redundancy coding may be selected to ensure reliable communication.
By dynamically adapting to the channel conditions, AMC systems strike a balance between data rate and reliability, enabling wireless communication systems to maintain a certain Quality of Service (QoS) even in challenging fading environments.
The impact of fading on wireless communication systems is substantial and multifaceted, affecting signal strength, data accuracy, and overall system performance. To address these challenges, communication engineers employ a combination of techniques, including diversity methods and adaptive modulation and coding, to mitigate the effects of fading and provide users with reliable and uninterrupted communication services, even in dynamic and challenging wireless environments.
Mitigation Strategies
Fading is a common phenomenon in wireless communication that can lead to signal degradation and data errors. To ensure reliable and robust communication, various mitigation strategies are employed. Here, we delve into these strategies, highlighting their importance in addressing the challenges posed by fading:
- Equalization:
- Channel Coding:
- Interleaving:
- Diversity Schemes:
- Space Diversity: Space diversity involves the use of multiple antennas at the transmitter and/or receiver. By receiving signals through different spatial paths, space diversity helps mitigate fading-induced variations. Techniques like Maximal Ratio Combining (MRC) and Selection Combining (SC) are employed to combine the signals received by multiple antennas to improve signal quality.
- Time Diversity: Time diversity, also known as time-domain diversity, involves retransmitting the same data over multiple time instances. By capturing the variations in the fading channel over time, time diversity reduces the probability of errors, especially in cases of deep fades.
- Adaptive Systems:
Equalization techniques play a crucial role in combating the effects of fading by compensating for signal distortion caused by multipath propagation. The primary objective of equalization is to recover the original transmitted signal, which may have been distorted during transmission through the fading channel.
Equalizers work by adjusting the received signal to minimize the impact of channel-induced distortions, such as intersymbol interference (ISI). By mitigating ISI, equalization helps improve the accuracy of symbol detection, reducing the Bit Error Rate (BER) and ensuring data integrity. Common equalization techniques include Zero Forcing Equalization and Minimum Mean Square Error Equalization.
Channel coding, specifically Forward Error Correction (FEC), is an essential component of fading mitigation strategies. FEC codes are designed to introduce redundancy into the transmitted data, allowing the receiver to detect and correct errors that occur during transmission. More robust FEC codes are used in severe fading conditions to provide a higher level of error correction.
By encoding data with FEC codes, wireless communication systems can recover lost or corrupted bits, thus improving the reliability of communication. Popular FEC codes include Convolutional Codes, Turbo Codes, and Low-Density Parity-Check (LDPC) Codes.
Interleaving is another technique used to address burst errors caused by fading. In fading channels, consecutive bits in the transmitted data stream are susceptible to being corrupted in clusters due to rapid signal fluctuations. Interleaving rearranges the order of data bits before transmission, ensuring that consecutive bits are spread out across the data stream.
By spreading errors in this manner, interleaving makes it easier for the channel decoder to correct them, as errors are less likely to be clustered together. This technique is particularly effective in scenarios where burst errors are prevalent, such as in fading channels.
Diversity schemes are fundamental to mitigating the effects of fading in wireless communication systems. These schemes leverage the use of multiple signal paths to improve signal reliability. Two common diversity techniques are:
Both space and time diversity enhance the reliability of wireless links by exploiting the differences in fading characteristics along different paths or over time.
Adaptive systems continuously monitor channel conditions and adjust their parameters in real-time to optimize performance. These systems employ adaptive modulation and coding (AMC) to dynamically select the most suitable modulation scheme and coding rate based on the current channel conditions.
In fading channels, the quality of the channel can change rapidly. Adaptive systems ensure that the communication system adapts to these variations by using higher-order modulation and lower coding rates when the channel is favorable, and lower-order modulation with higher coding rates when the channel deteriorates. This approach helps maintain a certain Quality of Service (QoS) even in challenging fading environments.
Conclusion
In conclusion, understanding the theoretical aspects of fading in wireless communication systems is essential for university students tasked with implementing MATLAB simulations or assignments related to this topic. We've discussed the fundamental concept of fading, explored different fading models (Rayleigh, Rician, Nakagami), and highlighted the impact of fading on communication systems. Additionally, we touched upon mitigation strategies that can be employed to enhance the reliability and performance of wireless communication in fading channels.
By grasping these theoretical foundations, students will be better equipped to tackle practical challenges in wireless communication system design and analysis, including MATLAB simulations and assignments that require a deep understanding of fading effects.